Since that time, hundreds of scientists have tried to understand the nature of cosmic radiation, and it is known a lot at the present time. It was shown that cosmic rays is an atomic nucleus ingoing uniformly from all directions (isotropic) of surrounding space of Earth. The most intriguing feature of this radiation was a power-law distribution of particles at energy I (E) ~ E-, extending by many orders of power from 106 to 1020 eV (the energy of the particles is usually measured in units of electron volts: 1 eV = 1.6 10-12 erg, GeV = 109 eV and 1012 eV = TeV units will frequently occur). Scientists were able to determine that, cosmic rays have mostly a galactic origin to an energy ~ 1018 eV (Galactic CRs - GCR). And CR coming from other galaxies to Earth begin to dominate at high energies. In addition, the estimated density of CR energy in the galaxy was infinite ~ 1 eV / cm3. It’s comparable to the total density of electromagnetic radiation of stars in the galaxy, the energy of the thermal motion of interstellar gas and kinetic energy of its turbulent motion and energy density of magnetic field of the galaxy. It allows to consider that cosmic rays are the most important component of interstellar medium of the galaxy. And the determination of CR sources and the study of its spread process to Earth are ones of the most important tasks in astrophysics.
The first attempts to explain the GCR origin were made from the energy balance of the Galaxy. Supernovae bursts, probably the most powerful energy sources in our Galaxy, have been considered as such objects the last few years. But despite the long history of GCR research, their origin (especially in the field of high energy) does remains a mystery. Many issues have not received the answers. What are the facilities and how to accelerate cosmic rays in excess of energies at many orders of magnitude, that can generate particles from the grand thermonuclear furnaces – the interior of stars? Is there a single forming mechanism of particle streams or several various mechanisms, acting in different areas of energy? What energies does cosmic rays of extragalactic origin begin to dominate? The fracture presence (the abrupt change of power-law spectrum, used to call the “knee”) at energy ~ 3x1015 eV has not explained till now. It’s one of the mysteries in the CR spectrum, discovered in 1958. Many hypotheses have been proposed to explain the whole complex of observed data of GCR, but the overall picture remains unclear and contradictory in many ways.
To some extent, unsolved issue of GCR origin is the fact that these particles are electrically charged and spread from the source to registration place not in a straight line, but changing its original direction, deviating in magnetic fields of the Earth, the Sun and the Galaxy. That’s way we can not to register the GCR of low energies in the near-Earth space. They reflected by magnetic fields of the Earth. But the source of high-GCR couldn’t be observed in the way like we see the star too. The original direction is distorted by magnetic fields of the Galaxy. For this reason, the “rays” name couldn’t be successful, because the flow of GCR particles does not spread in a straight line.
If we study the light from a star, we register the photon flux with quite low energy ~ 1 eV, but modern technology allows to record photons with very high energies up above 1012 eV (photons at high energies used to call gamma quanta). According to the laws of nuclear physics, gamma quanta with energies above 1012 eV = 1 TeV can formed by charged particles with energies on the order of magnitude above - 1013-1014 eV during their interaction with the interstellar medium. It means that the GC acceleration place of high energies could be seen. Science that studies the galaxy in the streams of high-energy gamma quanta, called the TeV’s gamma-astronomy. It was originated in the 70s of the last century, and it was able to solve the problem of CR origin, as at seemed that time. But it hasn’t happened so far. Although several sources in the Galaxy, which formed with TeV’s gamma quanta, have been found (the shells of supernovae and pulsars).
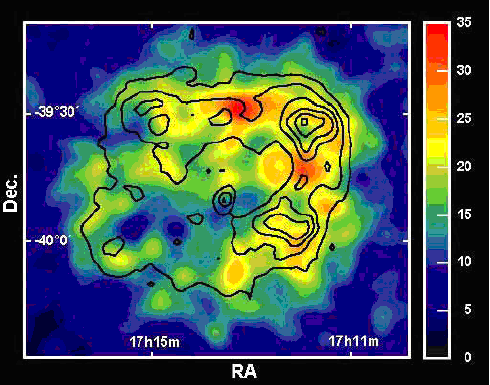
Possible acceleration place of CR spied by H.E.S.S. gamma-telescope (supernova remnant J1713.7-3946)
After analyzing the data from the N.E.S.S gamma-telescope – the most powerful at the moment, it has become clear that the CR origin problem is even more interesting than expected. For example, gamma- astronomy does not see sources of high-energy (> 1 TeV) gamma-quanta from all nearby supernovae remnants, but only from a small part, and the sources of gamma-quanta of MeV’s (1 MeV = 106 eV) and TeV’s energies are not the same (although the GCR range is power-law and continuous), and a variety of sources was very great. It remains to add that it was found several unidentified sources of gamma-quanta at a very high energy, including sources from our galaxy. The extended sources in the direction of the galaxy center have been discovered. These sources emit high-energy gamma-quanta and can not be seen either in the optical light or x-ray range. The authors called them "dark accelerators". That’s why it’s doubtfully that supernova is the only major CR accelerator in the galaxy.
The history of GCR research shows that only new experiments step by step, the installation by the installation, accumulate grains of knowledge about cosmic rays. It is highly may be that the mystery of CR origin still remains enigma, because we almost do not know anything about the fundamentals of matter and energy in the universe (dark matter and dark energy), as it has turned out in the last 10 years. Including the mass of dark matter in our galaxy, which is exceeds the known matter to us in many times over.
NUCLEON experiment is one of such experiments, aimed to measure the CR spectra in the area directly adjoining to the "knee" in the GCR spectrum. It will be shown below that the dependence of CR particles intensity from energy (CR energy spectrum) and the CR distribution on the charge and nucleus mass (chemical composition) contain important information about the CR sources, acceleration mechanisms and CR spreading in the galaxy.
The total energy spectrum of the GCR
Scientists faced with two major methodological difficulties in studies of GCR:
1. The primary flow of GCR does not reach the surface of the earth, even the surface of the highest mountains. Numerous interactions of particles and air atomic nucleus happen, as they entry into the dense atmosphere layers. Therefore, the study of GCR with "direct" methods (in which the energy and charge of the primary particle measured) is possible only outside the atmosphere, with the help of satellites or high-altitude balloons.